Nerve cells function as "inductors" (Mf p.
338) and contributes to govern the development of organs during
fetal stage. It seems quite natural with the general view
on nervous and nutrition systems as primary opposite vector fields
that it is the interplay between these fields that differentiate
organs. They are fields from the animal and vegetative poles of
the gastrula,
from 00- and 0-poles in terms of our model.
On the molecular level, the same peptides may
function as both transmitter substances in the nervous system and
as digestive enzymes.
As said about glands
the two vector fields meet and combine in hypothalamus with hypophysis
and adrenal glands with tissues from both fields. Information goes
there from the nervous signals to the chemical ones and blood stream
of the nutrition system, an expression for the first inward direction
of the nervous system (Ns).
The origin of Ns from the 00-pole and inward direction
seems revealed also in the fact that cortex of the brain primarily
is a development of the sensory system (olfactory brain) - i.e.
the inward directed signals.
The three kinds of stimuli, chemical →
electric → mechanical,
can be associated with matter →>
charge →> motions →>
in dimension degree (shortened d-degree) steps 3 →
2 → 1 →
0/00 in the dimension chain.
In the propagation of nervous, electric signals
the mediating chemical synapses can be interpreted as a binding
force, as in the dimension model higher d-degree in relation to
next lower one.
1. Polarizations within the nervous system:
The several polarities within Ns can be outlined in accordance
with the elementary physical
qualities interpreted as a dimension chain - with certain
connections to the chain of levels in an organism:
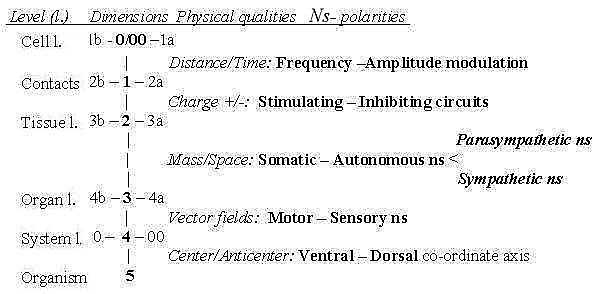
Fig Ns-1
First polarization, step 5 →>
4, refers to the animal and vegetative poles of the embryo, commented
above: Ns that develops from the animal pole (00) becomes the
front end, position for brain - as the spinal cord stretches
along the dorsal side - in opposition to vegetative pole,
becoming back end and ventral side.
- Step 4 →> 3 implies
the polarization of vector fields in inward - outward directions,
in Ns the sensory and motor systems.
- Step 3 →> 2 as a polarization
central - peripheral Ns follows mainly the differentiation
of organs (level 3) and tissues (level 2): muscles
versus guts, somatic versus visceral Ns. Cf. the interpretation
of muscles,
striated versus smooth ones as a polarization in step 3-2.
The polarization has features of both the preceding
ones; the opposite origins of organs and the outward /
inward directions.
Central Ns governs skeleton muscles, while the
peripheral Ns governs not least the walls of blood vessels and intestines,
walls as surfaces, and circumference of their inner space, an opposition
also of the character mass - space, interpreted as polarity in step
3 - 2.
Secondarily the peripheral Ns gets polarized in the sympathetic
and the parasympathetic Ns, an opposition which in function
is related with both the main directions outwards/inwards
and the next step: stimulation - inhibition.
- Step 2-1: Stimulation - Inhibition concerns charge
over nerve cell membrane: hyperpolarization or depolarization of
charge (proposed as a physical property of d-degree 2 in the model,
relative mass when analyzed as of degree 3). It gets expressed in
the design of different cell contacts in Ns.
- Step 1 →> 0/00:
Frequency - Amplitude modulation concerns the electric
signals of individual nerve cells and is connected with the elementary
physical concepts Distance (amplitude, ~ distance from a basic line)
and Time (1/f, frequency).
[The nervous system develops in similarity with other organs, e.g.
the blood system, from individual cells to threads, nets and layers,
through concentration of nerve cells to ganglions and via tube-shapes
to the centered structure of the brain: in shapes 0 →1 →
2 → 3.]
2. Motor - Sensory systems:
Direction between the organism as center and its environment as
anticenter is polarized in inward direction, sensory stimuli from
outside, and outward direction, motor stimuli from inside. These
main directions are also expressed in vertebra of the backbone,
where motor nerves depart ventrally while sensory nerves enter dorsally.
The 00-pole of the embryo becomes the dorsal side,
its 0-pole the ventral side. The fact that sensory nerves enter
from the dorsal side into the spinal marrow and that sensory areas
are located dorsally in neural tube and brain is hardly a matter
of course, sooner an expression of underlying dimensional rules.
The principle in a vertebra:
Dimension degree
4:
Directions out-in.
4 horns. |
Fig
Ns-2-73-1 |
In accordance with the same geometry sensory signals in "afferent"
fibers up to the brain pass through the posterior tract, i.e. along
the dorsal side, while the "efferent" motor ways go ventrally
in the anterior cerebrospinal tract.
Further, the switch-over stations in the sensory
system are situated in ganglions outside vertebra, while motor ganglions
lie inside in the spinal marrow: also a feature of the type anticenter
versus center.
(It could be observed that the ventral horns are
thicker, more massive than the dorsal ones.)
Outside the vertebra and spinal ganglions sensory and motor nerves
run together in shared pathways, which thus illustrate two-way direction
(as of not polarized, two-way directed d-degree 4). These branch,
as on a superposed level, in agreement with the same, underlying
fundamental polarity, to dorsal and ventral sides:
Fig
Ns-3-73-2
It's a remarkable circumstance too that cortex of the brain, front
end of the neural tube and secondary 00-pole of the embryo, develops
out of the sensory regions.
(The layer furthest out in cortex is also dendrites,
the inward conducting extensions of the nerve cells.)
The more ventral motor areas of cortex appear
to be of a secondary kind with mostly a regulating function in relation
to primary motor centers for movements deeper in the brain. This
is another illustration of the underlying polarity center (0) and
anticenter (00) and also to a certain degree of the polarity mass
- shell in the brain, d-degrees 3-2.
Sensory and motor nerve cells:
In the dimension model step d-degree 4 →>
3 is hypothetically connected with an angle step 180° to 90°,
where outward direction gives the radial component in d-degree 3,
the inward direction the circular component, in terms of elementary
geometries.
These geometries can be found in the difference
between motor nerve cells with axons, radially branched, outwards
from the cell, and the sensory pseudo-unipolar cell which has its
axon in straight angle to the cell:
Fig
Ns-4-75-1
Interneurons:
In this macrostructure of pathways the development of interneurons
and reflex arcs may be interpreted as a result of a polarization
towards a "perpendicular" relation in d-degree step 4
→> 3.
It can be noted too that the stretch reflex doesn't
have any interneurons, while the flexor reflex passes via several
interneurons. Cf. flexing, bending as a turn towards curved structure.
(Interneurons in the macrostructure of pathways
have a certain similarity with dendrites on the cell level in its
combining of signals from different directions - like an arc of
a circle passes though a multitude of angles and coefficients of
direction.)
Fig
Ns-5-75-2
The transition to circular structure and to rotation becomes most
obvious in the "reverberating" circuits, closed chains
of interneurons just in sensory, inward conducting pathways, where
the signals can rotate self-propelled (Nf p.108).
Fig
Ns-6-75-3
Number of steps in transport of a signal:
Generally there seems to be about 4 neurons in the shortest sensory
conductive pathways inwards to cortex of the brain, including the
neurons in cortex. 5 with the receptor cell (MF p. 360):
to compare with steps in a dimension chain 4 ←←←←
00.
Afferent pathways for sense of touch and proprioceptors:
→> 00: sensory receptor
→> 1: sensory neuron in
spinal ganglion
→> 2: switch-over
station in spinal marrow or in medulla oblongata
→> 3: thalamus
→> 4: cortex
Afferent pathways for sight and for hearing:
→> 00 receptor cells (cones
and rods) and in the inner ear the hair cells
→> 1: bipolar cells* (sight)
and from ear 1 nerve cell in a ganglion
→> 2: ganglion cell in
retina and from ear 2 neurons in brain stem
→> 3: thalamus, sight and
hearing
→> 4: cortex, sight and
hearing
(*Apart from 2 layers of horizontally coupled
cells moreover. See file Sight.)
A note about cranial nerves:
An invertebrate as the bristleworm has 6 pairs
of cranial nerves. An early species of chordates as cyclostomes,
whose brain already is divided in regions typical for vertebrates,
has 10 pairs. From reptiles on there are 12 cranial nerves:
Fig
Ns-7
3. Somatic - Autonomous (Visceral) nervous system:
Fig
Ns-8
The polarization into somatic and visceral, autonomous Ns concerns
directions in relation to governed organs in the body as a 3-dimensional
whole:
- the somatic Ns innervates striated muscles,
i.e. in direction outwards in the chain of organs,
- the visceral or peripheral nervous Ns innervate
heart as center of the blood system and the smooth musculature in
digestive canals, glands, blood vessels and so on, i.e. mostly organs
in directions inwards the body.*
The somatic Ns concerns external body posture
and movements and external locomotion, the relation to environment,
while the visceral Ns concerns the inner milieu of the body.
* The autonomous Ns innervates also such things as sweat glands
in the skin and e.g. the pupils.
Muscles
have in preceding files been proposed as derived in step 3-2 in
the level chain of systems (s):
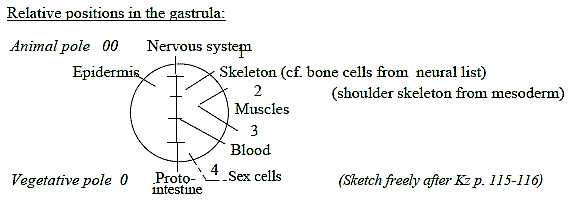
Fig
Ns-9
In the chain of organs the polarity somatic - visceral
muscles becomes a kind of border between "outward /
inward" directions in the middle step:
Fig
Ns-10
The visceral system as inward directed in the mentioned sense cooperates
with the sensory = inward directed system of the somatic one: visceral,
preganglionic nerves get activated by inward conducting afferent
nerves from both visceral and somatic organs
The autonomous system is in several respects secondary or "peripheral"
in relation to the central one (CNS) - as a lower d-degree in relation
to a higher one implies a further driven differentiation and a relation
of the type anticenter to center in the dimension chain:
The nerve cells in the sympathetic part of the
peripheral system, sympathetic ganglion cells and chromaffin cells
derive from the neural wall of the embryo, i.e. the anticenter to
the neural plate and invaginating neural tube (Kz p. 116).
Further, in the history of evolution the peripheral
system is weakly developed in early chordates as cyclostomes and
cartilaginous fishes, while it becomes more developed in bony fishes
(Fc).
It has also been shown that animals can manage
without the sympathetic Ns, although less well (Nf p. 344).
Intestines with origin from the vegetative 0-pole
are as such primary in relation to skeleton musculature (from mesoderm),
but from the aspect of the animal 00-pole the innervation of the
inner organs comes later than that of the skeleton muscles, in this
sense representing a later step.
Typical for the autonomous system is also that all motor pathways
go via intermediate synapses, in this sense act more indirectly.
In addition, the preganglionic motor neurons in
the autonomous system corresponds in their function to interneurons
in the central, somatic nervous system (Zf p.202), which
gives one more reason to see the autonomous (or "vegetative"
) system as a secondary development according to the interpretation
of interneurons above.
The position of visceral sympathetic neurons in the spinal chord
between dorsal and ventral somatic centers may perhaps also be an
expression for the secondary character of the autonomous system.
The autonomous system is to a great extent governed from hypothalamus
and the marrow of adrenal (suprarenal) glands,
organs out of the polar meeting between the nervous and the nutrition
systems as primary vector fields (4a →>
4b).
Hypothetically then the autonomous Ns could eventually
have a deeper root than somatic Ns as a "resting", potential
possibility, although developed later?
4. Sympathetic - Parasympathetic nervous systems:
The peripheral Ns polarizes in its turn in a corresponding way
as the polarization somatic - visceral Ns into directions outwards
- inwards in the body and also along the coordinate axis forwards
- backwards:
- the sympathetic system (SNS) is outward directed
in promoting outer activity, preparation for defense, activated
by stress and favors blood flows to skeleton muscles, heart, brain
etc.,
- the parasympathetic Ns (PNS) is inward directed
towards intestines, favors blood flows to the digestive organs and
depresses the heart activity etc.
Hence, the sympathetic Ns stimulates mostly organs
from mesoderm and ectoderm, outwards towards the 00-pole and environment,
the parasympathetic Ns mostly organs from endoderm, inwards the
0-pole, seen from the aspect of tissue origins.
Regarding the spinal cord as a coordinate axis between head and
tail, the parasympathetic nerves depart from the "outer"
poles, from head and sacrum, in this sense from anticenters, while
the sympathetic nerves depart from the central region:
It's hard to find any natural cause for this arrangement,
unless underlying dimensional aspects on directions are included.

Fig Ns-11-79
Parasympathetic ganglia have few mutual connections
and their effect is local, limited to one organ (Nf p. 343).
Cf. inward direction towards one 0-pole = towards one target. While
sympathetic ganglia are mutually united through the sympathetic
chains (or trunks) on each side of the spinal cord, and their effect
is more general and unspecified - as outward direction from a 0-pole.
Position of ganglia as stations for transmission illustrates the
same geometry, the typical center - anticenter relation: they lie
in the sympathetic Ns near the vertebra, in the parasympathetic
Ns further out, at the target organs as illustrated in the figure
above.
In pupil reflexes of the eye the complementary effects of S- and
P-systems show the radial versus circular polarity of d-degree 3
in the dimension model:
- the parasympathetic nerves go to the ring-formed
iris sphincter muscle for constriction of the pupil,
- the sympathetic nerves go to radial muscles
that widen the pupil.
It's perhaps the most typical illustration of the "postulates"
in the model: of inward direction, equivalent with contraction (convergence)
leading to circular structure in lower d-degree, and of outward
direction as divergence (widening), leading to radial structure
in lower d-degree.
However, both P- and S-systems have double effects
of widening - contraction, but mostly then divided on different,
more or less complementary organs.
Further, the P-system increases secretion of electrolytes, the
S-system increases secretion of organic substances (Mf).
This difference could be regarded from the aspect
of chemical phases:
organic molecules as a 3-dimensional phase versus fluids as a 2-dimensional
one with regard to bonds in the molecules. There is also a connection
with the concepts mass versus charge as a d-degree relation of type
3 to 2 in the fundamental chain of physical qualities.
Number of departing S- and P-nerves from neck and backbone in humans:
(According to a figure in Kz p. 257. Accidental or not?)
S = 18
P 5+3 = 8
The 2x2-chain behind the periodic system:
Fig
Ns-12-18-8
5. Stimulation - Inhibition:
This polarity concerns charge,
the quality that has been assumed defined in d-degree 2 in the dimension
chain of physical properties. It works through hyper- or depolarizations
over cell membranes. The quantity permeability as inversely proportional
to charge gets localized to different canals in the membranes (d-degree
2) for different ions. Stimulation occurs through inflow of Na+
ions, inhibition probably through inflow of Cl-
(Nf p. 111 f, 114). Hence, it would be a polarity between
charges of the ions (or size?), not of directions.
According to the loop version of a dimension chain
we could have a connection between the polarization motor →←sensory signals
in step 4 →> 3 and "the
other way around" the stimulating-inhibiting system in step
2 ← 1.
Fig
Ns-13-81
Inhibition is in several respects characterized
by features from the 00-pole - from anticenter.
In the history of evolution certain facts indicate that the polarization
first concerns the membranes of receiving cells, i.e. in
inward direction of the cells: the same transmitter, e.g. Acetylcholine,
can have inhibiting effect on one cell, stimulating on another.
It implies that the same sender cell can have activating or hampering
effect on different cells, so in certain mollusks (Nf p. 118
f). (With the postulate in the model that the 00-pole and inward
direction is the first polarizing force this circumstance could
be taken as another indication that dimensional polarities are underlying
the biochemical expressions for them.)
In mammals a division of functions is carried through so that certain
cells are inhibiting, other stimulating in their outward activity
- with different transmitters for stimulation and inhibition. It
could be interpreted as a substantiation of polar functions towards
superposed levels in accordance with the dimension model.
Another example is the polarization that seems
to occur in the brain during evolution between inhibiting and stimulating
nuclei as striatum and pallidum.
Inhibition is mediated via interneuron between sensory and motor
nerve cells (as if it were a polarization of the interval from sensory
to motor cell, cf. the preceding figure).
Geometrically it implies a development towards
circular structure and loops in the conducting lines S - M, dimensionally
as in steps 4 →> 3 →>
2,
Such structures polarize further into stimulating
and inhibiting interneurons.
The shortest, closed loop seems to be the self-inhibition of the
motor α-neurons
via interneurons (Renshaw-cells) to their own incoming signals.
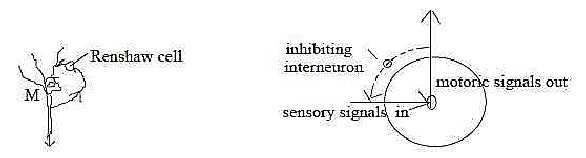
Fig Ns-14-82-1
The polarization of muscles into antagonists, such as flexor- and
stretch-muscles on opposite sides of limbs, seems expressed in mutual,
reciprocal inhibition between the antagonists via interneurons.
(Compare inside/outside as one geometrical
definition of poles of d-degree 2 in the model in arrangement of
muscles with stimulation - inhibition as a polarization in step
2 - 1.)
What is called "lateral inhibition" sideways exists on
all levels in the nervous system and is principally perpendicular
to in- and outgoing signals. (Cf. angle steps →>
180° →> 90°
,
associated with d-degree steps 4 →>
3 ...)
Fig
Ns-15- 82-2
It's said that there exist few connections between columns that
register different sensory types in cortex in the brain. Those that
exist seem to be inhibiting ones. Branches from pyramidal cells
in layer 5 go to star cells in layer 3 and 2, which sends inhibiting
threads to pyramidal cells in adjacent columns, i.e. sideways (Nf
p. 237, 254).
In a corresponding way purkinje cells in the cerebellum
inhibit one another via basket cells, whose threads are transversal
to the espaliers of purkinje cells (Nf p. 300).
The structure serves discrimination that implies
sharpening of contrasts, borders, lines: cf. surfaces, d-degree
2 and lines 1. So for instance in retina in the eye.
Fig
Ns-16-82-3
We can find a similar principle in the vegetative world, where
top shoots hamper the growth of side shoots through the substance
auxin.
Lateral inhibition appears not only in eyes but also in other senses
as in hearing and in skin (Nf p. 183): stimulation of the
skin around the domain of a certain nerve cell hampers the signals
from this. It's then an inhibition from anticenter inwards a center.
Fig
Ns-17-83-1
The inhibition between different kinds of sensory signals, i.e.
between qualities, was mentioned above. One example is that touching
can hamper signals of pain. Possibly however, this type could be
a question of positions too, since they concern the same domain
in the skin, although between different kinds of receptor cells. There
is reason to suspect that the different senses are differentiations,
mutually connected. (See about senses
with aspects from the dimension model.)
A primary type of inhibition, where signals from anticenter via
synapses hamper a motor signal from center is exemplified by the
sensory Golgi organs in tendons at insertions
of a muscle. (Cf. tendons as connective tissue on a tissue level
referred to d-degree step 2 - 1 in earlier interpretations here.).
Contraction of the muscle, implying stretching
of tendons, leads to inhibiting signals via interneurons
in the spinal chord to α-neurons
of the muscles (Nf p. 206 f):
Fig
Ns-18-83-2
Muscle spindles, in the center of the muscle, are
much more complex and directions of signals from center and anticenter
the complementary ones: Outgoing (afferent) from the center of the
spindle, to alpha-neurons in spinal chord, while incoming signals
(efferent, from gamma-neurons) go to the ends at anticenters of
the spindle. The central part of these fibers are not even contractile,
only the ends at anticenter. Cf. contraction as directions inwards
from 00-poles, outwards from 0-poles.
The inhibiting function of the anticentric gamma-neurons
seems not yet fully understood but is expressed as effecting the
sensitivity of the spindle (Nf, Aph, Mf).
With the coordinate axes of the body in mind, Front - Back from
Animal - Vegetative poles, there are several examples showing that
inhibiting signals originate from the 00-pole or from secondary,
superposed levels, which also as such represent anticenter in relation
to underlying ones.
Fig
Ns-19-84
We have the already mentioned example that motor areas in cortex
mostly have the function to regulate sensory inflow and therewith
indirectly modulate motor outflows (Nf p. 264-265). While
the essential stimulation to movements comes from inner centers
in the brain and brainstem, from there to cortex and back.
Inhibiting impulses from cortex have disappeared
at spastic movements and released exciting impulses from the reticular
formation and vestibular nuclei in the brainstem (LEL p. 133).
The motor pyramidal pathways that go from cortex
in the brain directly down to the spinal cord are physiologically
younger than the other "extrapyramidal" pathways from
inner centers in the brain, and they seem mostly to have a function
to regulate distal fine motor ability (Fz p. 355). A big
part of them go to interneurons from sensory spinal ganglia
in the dorsal horns of the spinal cord.
The pyramidal pathways can be cut off without
loss of movability, not even loss of movements governed by the will.
Only precision and velocity become weaker and slower (LEL p.
160).
In cerebellum (note its dorsal ~ anticenter location) the inhibition
processes are dominating, while pathways for stimulation come from
inner nuclei in the brainstem (Fig. Nf p. 282).
The polarity stimulation - inhibition can be regarded also as a
specialization of the underlying polarity within the autonomous
system in sympathetic - parasympathetic polarity, concerning stimulation
- inhibition of blood flows to different organs.
According to certain observations (1978) inhibiting transmitters
should lie in elliptic granules in the ends of axons, stimulating
ones in round granules (Nf p. 117). Ellipses are polarized
circles with two centers. Hence, also in such a detail, if the observation
is correct, we could find a trait of the 00-/0-polarity.
6. Frequency - Amplitude modulation:
Amplitude and frequency are coupled entities in a sine wave, complementary
energy forms as potential energy and kinetic energy. Potential energy
= distance from a zero-line as 0-pole, kinetic energy passage through
the zero-line per time unit. Hence the quantities are connected
with distance and time respectively and this polarity is suggested
as last step in polarizations within the nervous system.
Fig
Ns-20-90-2
In an atom the two energy forms are transformed into one another
at absorption and re-emission. The amplitude of an electron orbit,
distance from the nucleus, increases at absorption of radiation
(inward direction) and becomes a measure of its energy. It translates
into frequency of emitted radiation (outward direction) when the
electrons fall back again to an inner orbit, the frequency depending
on radial distances between different orbits.
In the nerve cell there is the same principle: incoming chemical
signals become amplitude modulated in the cell membrane; outgoing
electrical signals in the axons become frequency modulated.
Geometrically it illustrates the poles circular
- radial structure out of d-degree 3 in step 3 - 2 in the model:
circular structure from inward direction connected with amplitude,
radial structure from outward direction connected with frequency.
(From the electrons point of view the description
can seem reversed: outward jumps defining amplitudes,~ distances,
d-degree 1, inward jumps giving the frequency, ~1/Time.
Thus, we have a kind of pole exchange out/in
between electrons and EM-waves as assumed in "d-degree 0/00"
of motions in our model.)
To Nerve cells and the nervous impulse
To Nervous system: Brain parts
|